Challenge–Inspired Polymer Synthesis
Modern society faces an array of challenges. New treatments for disease, sources of energy, and greener materials are in demand. These challenges also reveal interesting scientific questions, which as scientists we seek to answer. The interplay between investigating “challenge-revealed” fundamental questions and synthesizing “challenge–inspired” materials characterizes the overarching philosophy of our research approach.
Glycomaterials for Antibacterial and Antiviral Applications
We are working on developing antibacterial and antiviral glycopolymers—polymers that are appended with bacteria- or virus-binding carbohydrates capable of inhibiting infections. Bacterial and viral infections are commonly treated with vaccines, antiviral drugs, or antimicrobials; however, there are limitations to this approach (e.g., strain resistance or specificity) that require the development of novel antiviral and antibacterial materials to circumvent such restraints.
Work on this project is broken down into 2 main research interests:
- Synthesizing carbohydrates that both mimic the glycans found on cell surfaces and contain linkers to connect them to polymers. Glycans of interest range from monosaccharides like sialic acids and mannoses to more complex structures such as histo-blood group antigens.
- Polymer synthesis of modular polymers with different architectures (i.e., linear, hyperbranched, star) or backbone identity (i.e., polypeptides) to investigate structure-property relationships
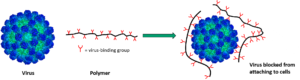
Polymers for Extracting Critical Metals from Complex Solutions:
f–Block elements are critical to the technological and energy infrastructure that underpin both our modern and future worlds, but as global demand for these metals outpaces supply, new and sustainable sources are needed, as well as more efficient methods of extraction and purification. Metal–binding polymers are an emerging class of materials that have the potential to improve or supplant existing processes for extracting metal ions from solution.
We use isothermal titration calorimetry (ITC), a technique widely used to study the binding of biological molecules, to directly determine the impact of all aspects of polymer structure on the underlying binding thermodynamics. We are interested in how the polymer structure and topology play a role in metal–binding interactions, beyond and in addition to the chelating group identity. We are also working to develop new ITC methods to study polymer–metal interactions in a variety of relevant environments. These results lead directly to new design principles that will improve materials used in environmental remediation applications—including mining waste processing and water treatment—as well as extraction and purification of scarce metals from (un)conventional sources.
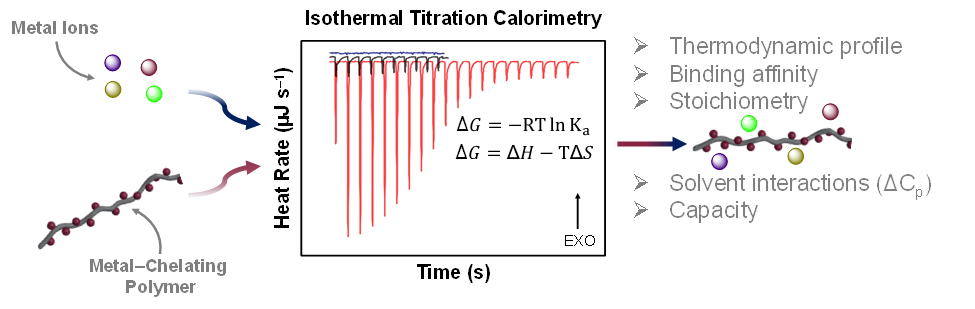
Novel Polymers and Polymerization Catalysts:
Advances in fundamental polymer chemistry lead to new material properties. Consequently, we are interested in developing polymers with unique structures and functionalities. For example, we are developing novel polyesters with high glass transition temperatures, UV-stability, and favorable barrier properties. Additionally, we are designing new olefin metathesis catalysts that are tailored to create polymers of specific architectures. The materials that result from these efforts will both provide key insights into polymer structure-property relationships and enable new applications to be developed.
Materials in Medicine:
Chemofilter:
The adverse side-effects of chemotherapy are a notorious problem in the treatment of cancer. One approach to mitigating these toxic effects, particularly in liver cancer, is transarterial chemoembolization (TACE), a procedure in which chemotherapy is introduced via catheter directly into the tumor vasculature. Despite this site-specific delivery, however, about half of the chemotherapy dose passes through the tumor, enters systemic circulation, and causes off-target damage. To address this problem, we are developing materials that are capable of capturing this residual chemotherapy from the bloodstream. Ultimately, these materials will be used to construct a device (a “ChemoFilter”) that can be depolyed via catheter “downstream” from the tumor, enabling excess chemotherapy to be intercepted and sequestered before it enters healthy tissue and causes side-effects. This is a multidisciplinary effort sponsored by the National Cancer Institute (R01CA194533; principal investigator, Steven W. Hetts) involving investigators at multiple institutions.
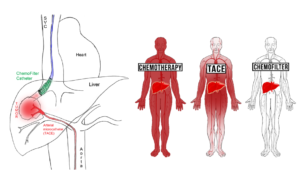
Surgical Sealants:
Tissue sealants are an important surgical tool—they are frequently utilized to heal ruptured dural sacs, a common complication that occurs during spinal surgery and causes cerebral spinal fluid leakage. The damage can be minimized by using the sealant to reseal the ruptured sac. The challenge of making an effective dural sealant is twofold. First, the sealant must be sufficiently hydrophobic to repel CSF. Second, the material must adhere to the dura with sufficient strength to resist CSF leaks over time. These two attributes are often in tension, however, as highly hydrophobic materials often do not strongly adhere to the dura. Beyond these two critical properties—CSF resistance and strong adhesion to dura—an ideal dural sealant must also be easy to use, biocompatible, biodegradable (on an appropriate timescale), and have adequate mechanical properties (strength, elasticity, etc.). Our goal is to design these novel hydrogels and investigate their potential as stronger tissue sealants.
Thank You to Our Sponsors!
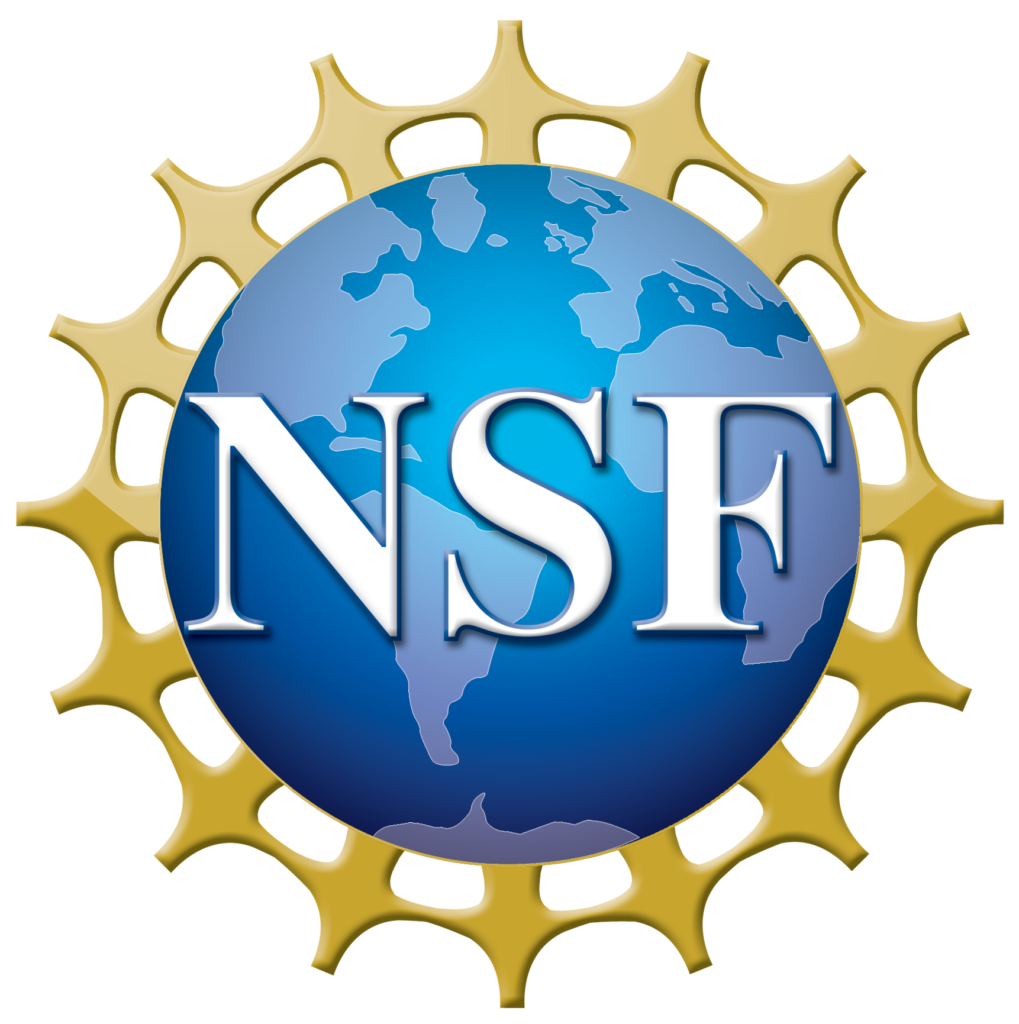
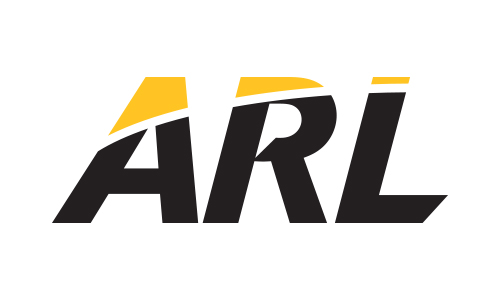
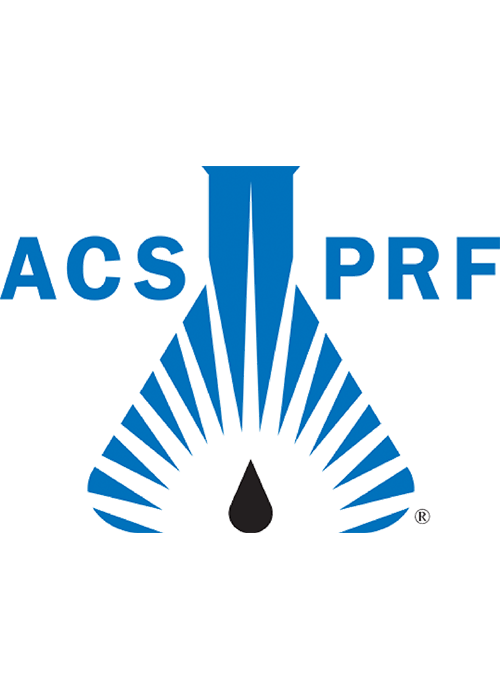
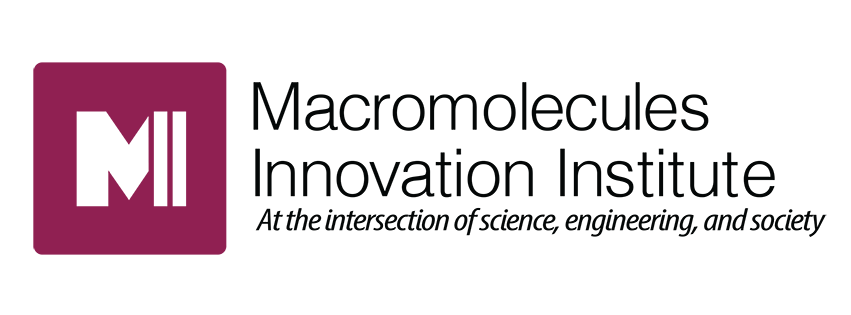
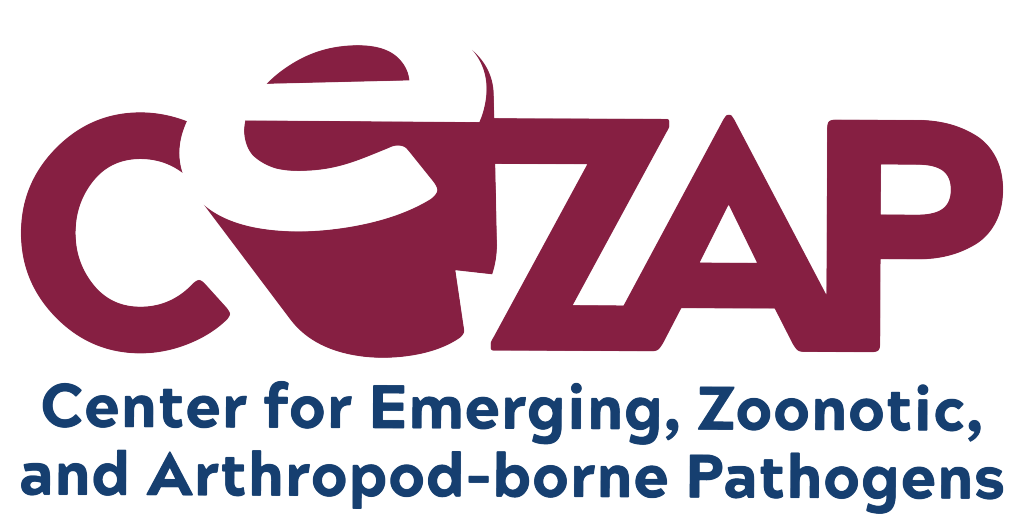
Virginia Tech Center for Drug Discovery
Industrial sponsors
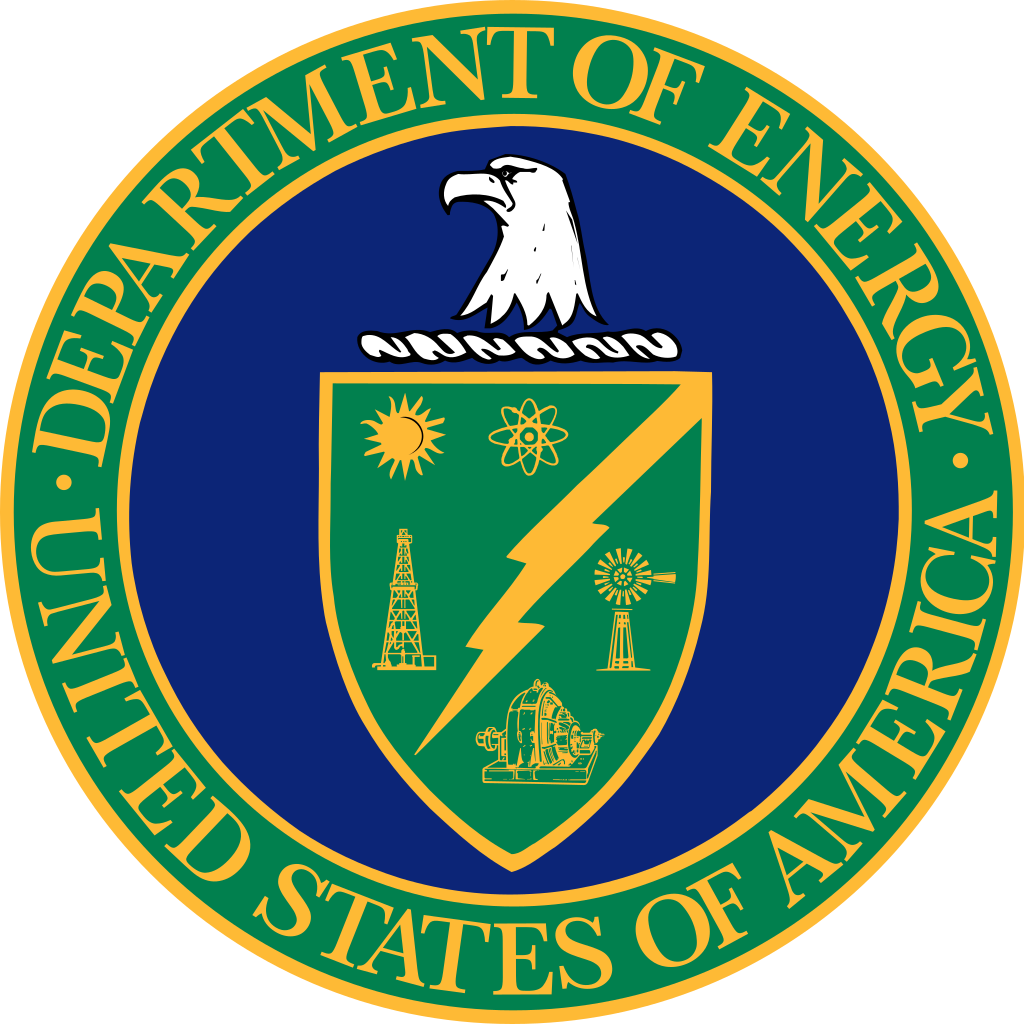